Researchers ID genetic underpinnings of modern tomatoes
UGA researchers have helped to unlock the mystery of what separates today’s tomatoes from their inedible ancestors
UGA researchers help identify ‘the mother of peanut’
While Americans are familiar with one or two varieties of peanut, farmers in other parts of the world have been able to develop hundreds of varieties thanks to the peanut’s natural ability to shuffle its two distinct subgenomes to produce new traits. These are some of the peanuts grown by the Caiabí people who live on the Ilha Grande, Mato Grosso, Brazil. Peanut is very important for them and they cultivate diverse types, each one with its use, name and story. (Photos by Fábio de Oliveira Freitas)
By J. Merritt Melancon
Working to understand the genetics of peanut disease resistance and yield, researchers led by scientists at the University of Georgia have uncovered the peanut’s unlikely and complicated evolution.
Researchers working as part of the International Peanut Genome Initiative have previously pinpointed one of the peanut’s two wild ancestors and shown that the peanut is a living legacy of some of the earliest human agricultural societies in South America. Since then the team has mapped the entire peanut genome and identified the crop’s second wild ancestor and the novel mechanism by which the shy, seed-hoarding plant generated the diversity we see today.
“Because of its complex genetic structure sequencing peanut was only possible using very recent developments in sequencing technology. The result is of unprecedented quality, and provides a reference framework for breeding and improvement of the peanut crop, and a whole new set of insights into the extraordinary genetic structure of peanut” said David Bertioli, Georgia Research Alliance Distinguished Investigator and peanut researcher at the College of Agricultural and Environmental Sciences.
Bertioli conducts his research through the CAES Institute for Plant Breeding, Genetics and Genomics, which is home to some of the world’s foremost experts in this area of crop science and has been prolific in providing new genomic tools and information to help plant breeders around the world develop more sustainable, productive crop varieties.
The team’s most recent paper was published in the journal Nature Genetics and is available online.
According to the USDA, farmers around the world grow 44.9 million metric tons of peanuts on more than 64 million acres. The crop is a staple food in many parts of Africa and Asia and is a source of peanut butter, snacks and cooking oil in the United States. In Georgia alone farmers grow $825 million in peanuts each year.
Despite their importance as a crop, plant researchers haven’t had many of the genetic tools needed to speed the introduction of more sustainable and productive peanut varieties. That was because, until recently, scientists had been unable to map the peanuts’ hypercomplex tetraploid genome. The Peanut Genome Initiative’s international collaboration and advancements in technologies and data processing yielded the breakthroughs.
Peanut genome sequenced
The bedrock of the team’s discoveries was the sequencing of the genome. Because the peanut originated from the hybridization of two wild ancestral species thousands of years ago, the initial phases of the project involved researchers developing genome sequences for those ancestors. Together, the ancestral genomes made a prototype for the genetic structure of cultivated peanuts. This was published in 2016.
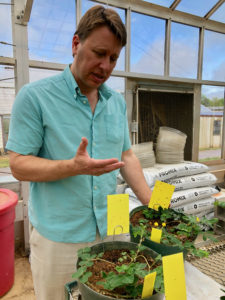
This month, the Peanut Genome Initiative discusses the entire genome sequence for the modern cultivated peanut in a paper published in Nature Genetics on May 1.
The researchers used new advances in DNA-sequencing technologies to produce a complete genome sequence of unprecedented quality. The sequence consists of more than 2.5 billion base pairs of DNA arranged in 20 pairs of chromosomes, 10 pairs from each of the ancestral species.
The information in the sequence sheds light on parts of the plant’s genetic code that control traits like seed size and disease resistance, which are important to plant breeders. But the sequence also revealed more about the origin of peanut during the dawn of agriculture in South America and on the genetic mechanisms that have generated diversity and allowed adaptation to environments around the globe.
The mother of peanut
Using the new genome sequence as a framework, the team was able to analyze the variations in more than 200 of the most diverse peanuts from all of over the world. Researchers found characteristic genetic fingerprints shared by all the peanut plants tested, providing new evidence that all modern peanut varieties stem from the same original hybrid.
“The new study underlines how peanut’s origin was due to very special circumstances thousands of years ago. Ancient farmers transported one species into the range of another, allowing their hybridization and the formation of a new crop species,” said Soraya Leal-Bertioli, a senior research scientist with the UGA Institute of Plant Breeding, Genetics and Genomics and the CAES department of plant pathology.
Scientists with the initiative had previously found the male donor of the original hybrid and origin of peanut’s “B” subgenome. In this new study, they identified the female donor, tracking the population of wild ancestral peanut that contributed the peanut “A” subgenome in Rio Seco, Argentina. These individuals form the “mother” population of peanut.
But the evidence that all modern peanuts can be traced to a single original hybrid sets up another mystery, Leal-Bertioli said. How does a plant with such a narrow genetic base develop so many variations and varieties?
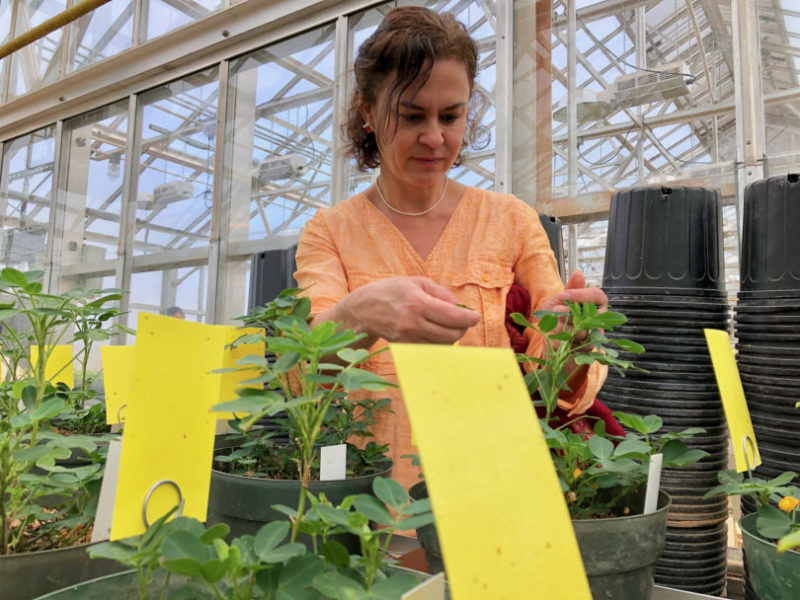
“Shuffling, shuffling”
Most flowering plant species rely on animals or weather to spread their pollen or seeds to other plants to generate genetic diversity. Pollen and seeds can travel for miles, spreading newly occurring traits to new populations.
But peanuts, which produce their seeds underground, don’t do that. It took early human farmers and their long-distance transport of seeds to get the first two ancestral peanut parents together.
Since then, however, the plant has used a new mechanism for creating diversity.
The peanut has two sets of chromosomes, one from each ancestor. By analyzing more than 200 cultivated peanuts from all over the world, it was shown that different landraces and cultivars have shuffled the genetic material of the ancestors and deleted some sections altogether. Over the past 10,000 years, this shuffling has happened thousands of times—allowing a much faster-paced generation of diversity than if the plants simply relied on mutation.
In a greenhouse on the UGA campus, the Bertiolis have worked with hybrids that re-create the original ancestral peanut and observed the shuffling in real time. They documented its effects in the spontaneous appearance of different flower colors. These same genetic mechanisms generate other types of variation as well, said David Bertioli.
The phenomenon explains the tremendous about of diversity seen in peanuts today, said Leal-Bertioli.
For this research, the International Peanut Genome Initiative brought together scientists from the U.S., Argentina, China, India, Japan and France. The initial sequencing was carried out at the Hudson Alpha Institute, Huntsville, Alabama, and USDA Agricultural Research Service Genomics and Bioinformatics Research Unit in Stoneville, Mississippi. The project was funded by the National Peanut Board, the American Peanut Shellers Association, and other growers, shellers, manufacturers and allied industries.
UGA scientists create world’s first gene-edited lizards
Lizard develops albinism traits through CRISPR gene manipulation
New comparative study on DNA modifications across the fungal tree of life
Scientists have published a study comparing and relating DNA methylation across 40 diverse fungal species
UGA to host NIH Compound ID Development Core
Identifying metabolites can help researchers understand human disease or provide the basis of drug discovery
UGA researchers look to speed up sustainable crop innovation with help from bladderwort
Plant’s unique genetic structure may shed light on ways to reduce crosstalk between new genes
The science of shape
One genetic mechanism is responsible for controlling the shape of tomatoes as well as the length of potato tubers, scientists say
Researchers discover how corn breaks genetics laws
Many heirloom varieties contain a cheater called Abnormal chromosome 10
New breeding method using plant’s own DNA could improve crops
Introducing a human protein allows researchers to selectively activate silenced genes
Sequencing the rice genome
Results will help plant breeders incorporate resilience traits from wild rice species into cultivated varieties
Sequencing the rice genome
Results will help plant breeders incorporate resilience traits from wild rice species into cultivated varieties
UGA to lead NSF project to rapidly sequence corn pangenome
Project will sequence genetic diversity of world’s largest cash crop
Size matters
The gene variants that control a tomato’s size have been found by UGA geneticists, who published their findings in the open-access journal PLOS Genetics.
Esther van der Knaap has spent much of her career working to understand the genetic shifts that occurred between ancestral, wild tomatoes and the modern, cultivated varieties that we eat today. Now, van der Knaap has helped to pinpoint a gene that regulates the size of the tomato’s individual cells, which in turn helps to regulate the size of the overall fruit.
“Knowledge of the gene will open up avenues of research into how fruit size can be increased further without negatively impacting other important qualities such as disease resistance and flavor,” said van der Knaap, a professor in UGA’s department of horticulture and Institute of Plant Breeding, Genetics and Genomics.
Van der Knaap and her colleagues investigated a gene they named Cell Size Regulator, or CSR, that increases fruit weight by enlarging the individual cells in the fleshy part of the tomato. Unlike wild tomatoes, domesticated varieties carry a mutation in the CSR gene that affects the way tomato cells develop before they ripen and fall off the plant.
The variation originated in the cherry tomato but now appears in all large cultivated tomato varieties.
This brief appeared in the fall 2017 issue of Research Magazine. The original press release is available at http://news.uga.edu/releases/article/taking-tomatoes-from-tiny-to-tremendous/.
Researchers publish first sunflower genome sequence
New resource will provide roadmap for more resilient crops
Stem cell treatment may restore vision to patients with damaged corneas
Researchers with UGA’s Regenerative Bioscience Center have developed a way to identify and sort stem cells that may one day allow clinicians to restore vision to people with damaged corneas using the patient’s own eye tissue.
Old into new: Geneticists track the evolution of parenting
The burying beetle is intimately involved in raising its children, including regurgitating food to its begging offspring.
Genetics faculty member honored with Presidential Early Career Award
University of Georgia assistant professor Andrea Sweigart is among 102 scientists announced as recipients of the Presidential Early Career Award for Scientists and Engineers.
Researchers explore and exploit bacterial immune systems
NIH grant supports study of CRISPR-Cas, a powerful gene editing tool derived from a defense mechanism evolved in bacteria and other single-celled organisms.
Building a better tomato
Esther van der Knaap joined the University of Georgia last year as part of a presidential hiring initiative to recruit extraordinary research faculty. A professor in the department of horticulture and the Institute of Plant Breeding, Genetics and Genomics, van der Knaap explores the genetic mechanisms underlying the formation of fruits and vegetables like tomatoes and peppers. She also studies the evolutionary processes that led to the domestication of these crops. Here, she discusses her work and how the genetic tools used in her laboratory may help boost the yield and quality of agriculturally important crops, and what the future holds for plant genetics.
You are widely recognized for your work on tomatoes. What drew you to this field of study?
I actually didn’t begin with tomatoes; my doctoral work focused on rice. I worked on a type of rice that grows very fast when the rice paddies are flooded during monsoon seasons, and I was studying the molecular processes that regulate their growth. I got a number of interesting leads from that study, but I didn’t have any way at that time to show that my leads were meaningful because of the lack of genetic tools. So that’s when I decided to start working on genetics. I didn’t really choose to study tomatoes per se; I had a really great opportunity to join a research program to do genetics research on tomatoes as a postdoctoral researcher, and I haven’t switched fields since.
What can we learn from studying tomato genetics?
Over the course of several thousand years, all agricultural crops and animals underwent a process called domestication where wild plants were adapted for human consumption. For tomato, this led to changing the fruit from a tiny round product to the large and variably shaped tomato that we all recognize. One thousand years may sound like a long time, but it is very recent on an evolutionary time scale. Because tomato domestication happened so recently, we can make a cross between a large tomato that you might buy in the grocery store and its closest wild relative and get very healthy seedlings with fruit that I can study further. That allows us to trace the genetic basis of the shape and size variation by mapping the various regions on its genome that control those traits.
How often does that kind of experiment lead to a new discovery?
Almost every time we identify a gene, we discover something unexpected. Sometimes we don’t know what the gene encodes, so our research leads to discoveries about genes that we don’t know very much about in plants in general. Sometimes we uncover unusual changes in the plant’s genome that gives us new information about how genomes evolve. It’s unpredictable. When we set out to identify another gene that controls the size of the fruit, I don’t know what we’re going to get, how long it is going to take and what we may do next. So a lot of our work focuses on basic scientific discovery. Yes, we are interested in the roles these genes play in controlling tomato shape and size, for example, but the genes we identify in those plants often help us better understand how they function more broadly in the plant kingdom. The potential for discovery really is endless.
Do these discoveries have any practical applications?
Absolutely. Our discoveries allow plant breeders to select seedlings with fruit traits that breeders want: large or small; oval, round or flat. We know, for example, that if a breeder wants to create slightly larger tomatoes in their lines, we can determine what crosses could be made to create a larger fruit by looking at their genotypes. If a breeder is working on a grape tomato and wants to create the same shape and size, but also improve it for disease resistance or some other quality, we know pretty well how to select for a type that will grow the right shape of fruit.
There has been a lot of public discussion about genetically modified plants. What are your thoughts about this debate?
All vegetables and fruits have been artificially selected by farmers since the dawn of agriculture. The genetic work we do in our laboratory leads to knowledge that can be implemented in tomato and other crops. By inserting a modified version of the gene we think controls fruit shape or size, we are able to prove the discovery of the gene that confers the expected trait. We need to make better progress on crop improvement because at the current rate we will not be able to feed the growing world population in the future. I hope that society will become more accepting of genome editing and plant transformation technology. In the future, a lot of our agriculture will rely on smart genetic modifications that make our food crops more resistant to pathogens or more resilient to climate change.
UGA geneticist studying neural tube defects for clues to common birth defects
An assistant professor of genetics at the University of Georgia was recently awarded a grant from the National Institutes of Health to study neural tube defects in mice. The goal of Jian-Fu Chen’s project is to understand why neural tube defects, the second most common birth defect in humans, occur.
Silencing of gene affects people’s social lives
A team of researchers led by psychologists at the University of Georgia have found that the silencing of a specific gene may affect human social behavior, including a person’s ability to form healthy relationships or to recognize the emotional states of others.
The CRISPR revolution
Scientists are buzzing over a recently developed gene-editing tool commonly known by the acronym CRISPR, which makes it possible to snip out and replace segments of DNA inside the cells of living organisms with extraordinary precision.
Babysitting bugs
May 10, 2016
“Burying beetles” are the undertakers of the animal kingdom. True to their name, these winged insects scavenge the landscape in search of small-animal carcasses, which they bury under the soil to save as a food source while they care for their offspring.
This beetle’s most unusual behavior isn’t the burying but the nurturing. While most insects do not care for their young, burying beetles do it to an extraordinary degree. They prepare food, protect the brood from invaders and even feed their begging young in much the same way that a bird feeds its hatchlings.
Now, a research team including scientists from UGA has identified many of the genetic changes that take place in burying beetles to help them assume the role of parent. Their findings, published in the journal Nature Communications, may provide clues about the fundamental genetics of parenthood in insects and other animals.
“Parenting is a complex trait, but it’s particularly complex in burying beetles,” said Allen Moore, a Distinguished Research Professor of Genetics in UGA’s Franklin College of Arts and Sciences and paper co-author. “Sometimes the male or female may care for the brood as a single parent, and sometimes they appear to work as a couple. By examining changes in genes associated with parenting, we got a clearer picture of what was happening.”
The researchers allowed 269 pairs of male and female beetles to co-mingle in a closed environment complete with food sources, and they observed the insects’ behavior as they raised their offspring. About 50 percent of the time, a male would abandon the nest after mating, leaving the female to care for the brood alone. Females, on the other hand, abandoned the nest only 5 percent of the time.
Regardless of its gender, when a single parent cared for the young, the researchers found that this beetle expressed specific genes that control parenting behaviors. But when the male and female appeared to act as a couple, the story was different.
“If you just observe a pair of beetles, they may look like they’re working as a team,” Moore said. “But our genetic analyses showed that the males really weren’t doing much of anything. They did not express the genes necessary to care for offspring, so the females were actually doing most of the work.”
Males and females may work together to bury a carcass and prepare the nest. But once their eggs hatch, the duties of parenthood generally fall to one just one of them, and that’s usually the female, Moore said.
While these discoveries will help scientists better understand the social dynamics of burying beetles, Moore and his colleagues hope to apply their approach to studying the behavior of other animals. “We now know which genes are turned on and off while these beetles raise their young,” he said. “Next, we want to see if those same genes influence social interactions in other species.”
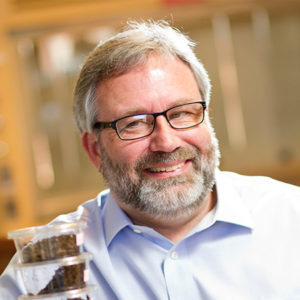
Allen Moore is a Distinguished Research Professor of Genetics in UGA’s Franklin College of Arts and Sciences.
Researchers trace peanut crop back to its Bolivian roots
Researchers at the University of Georgia, working with the International Peanut Genome Initiative, have discovered that a wild plant from Bolivia is a “living relic” of the prehistoric origins of the cultivated peanut species.
UGA scientists using $5 million grant to combat invasive weed
By James Hataway
A team of researchers led by faculty at the University of Georgia have received a $5 million grant from the U.S. Department of Agriculture to find new ways of combating Johnsongrass, one of the most widespread and troublesome agricultural weeds in the world.
Native to the Mediterranean region, Johnsongrass has spread across every continent except Antarctica. It was introduced to the U.S. in the 1800s as a forage crop, but it quickly spread into surrounding farmland and natural environments, where it continues to cause millions of dollars in lost agricultural revenue each year, according to the USDA.
“Weeds like Johnsongrass are a major challenge for agricultural producers around the world,” said Andrew Paterson, Regents Professor, director of UGA’s Plant Genome Mapping Laboratory and principal investigator for the project. “To make matters worse, widespread adoption of herbicide-resistant crops has been associated with a dramatic increase in herbicide-resistant weeds. With 21 genetically similar but different types of Johnsongrass known to be resistant to herbicides, it will only become more problematic in the future.”
Apart from its resistance to herbicides, the naturalization of Johnsongrass across much of the U.S. has also allowed the plant to develop attributes—such as cold and drought tolerance, resistance to pathogens and the ability to flourish in low-fertility soils—that make it particularly difficult to control.
Over the course of their five-year project, the researchers will work to develop a better understanding of the weed’s capabilities and the underlying genes that make Johnsongrass so resilient.
This information may lead to new management strategies that target and curb its growth, providing farmers with a more robust toolkit to combat the invasive plant.
But the researchers also hope that learning more about the fundamental structures that give Johnsongrass its unusual resilience will pave the way for new genetic tools to improve useful plants, such as sorghum, a close relative of Johnsongrass that is grown widely for food, animal fodder and as a source of biofuel.
“The close relationship between sorghum and Johnsongrass poses both a challenge and an opportunity,” said Paterson, who is housed in the College of Agricultural and Environmental Sciences and the Franklin College of Arts and Sciences. “The two species are so closely related that no herbicides distinguish between them, making control of Johnsongrass in or near sorghum fields especially difficult.
“Regardless, the lessons we learn from Johnsongrass may lead to strategies to improve sorghum and other major crops.”
Other researchers working on this project include Jacob Barney, Virginia Tech; Jeff Dahlberg, University of California Division of Agriculture and Natural Resources; C. Michael Smith, Kansas State University; Wesley Everman, North Carolina State University; Marnie Rout, University of Texas, Temple; and Clint Magill and Gary Odvody, Texas A&M University.