High-tech helps plan ahead for road improvements
Technology looks beneath roadway surfaces to assess their condition
Building Bridges
The multidisciplinary Institute for Resilient Infrastructure Systems is seeking innovative solutions to state and national problems ranging from flood control to sanitation.
In search of a cleaner, more efficient combustion engine
Emerging low-temperature combustion technology promises better fuel efficiency and less pollution
Scientists develop technology to capture tumor cells
Microfluidic device may help researchers better understand metastatic cancer
UGA researcher leverages artificial intelligence and social media to clean up recycling
Katherine Shayne has developed a system to educate consumers on what can or cannot by recycled in their area and how to do it properly.
UGA researcher leverages artificial intelligence and social media to clean up recycling
Katherine Shayne has developed a system to educate consumers on what can or cannot by recycled in their area and how to do it properly.
Engineering students showcase design solutions
College of Engineering’s annual Senior Design Showcase will be April 23
UGA New Materials Institute awarded Walmart Foundation grant
The $800,000 grant will aid in plastic packaging reduction research
Space Dawgs
Caleb Adams (left) and Graham Grable are part of the team that helped build this CubeSat and launch UGA’s Small Satellite Research Laboratory. (Photo by Dorothy Kozlowski)
By Aaron Hale
Caleb Adams (BS ’18) was looking for the next challenge. He and a group of friends—self-described geeks—had just created a smartphone-controlled telescope and launched a company to sell it.
He landed on cube satellites, or CubeSats, a class of miniature spacecraft shaping the next stage of space technology. CubeSats are compact and fairly inexpensive, at least compared to large communications and research satellites. Because they’re small and cheap, and because there have been huge strides in fitting high-powered computers into tiny devices, CubeSats are tissue box-sized vessels for innovation. Adams remembers thinking, “How hard can it be?”
Perhaps it’s fortunate he didn’t realize that competition for CubeSats is fierce, with universities vying for the limited approval and academic funding available to send a small satellite into space. But Adams and his friends aren’t easily daunted.
“I was looking for the most difficult thing I could find and throwing myself at it,” says Hollis Neel (BS ’17). “That’s a theme you’ll find with a lot of us: We really enjoy a challenge.”

As it turned out, faculty in the Franklin College of Arts and Sciences geography department had already been exploring CubeSats. Associate professor Deepak Mishra and research scientist David Cotten are monitoring the coastal water quality and wetland health around Georgia’s Sapelo Island. They are tracking algae blooms, sediment and organic matter in the water—all vital indicators of coastal and wetland health.
In addition to gathering data on the ground, the researchers verify their findings with visual information gleaned from large research satellites operated by NASA and the European Space Agency. But the satellites have set overpass times, usually going over Sapelo Island just a few times a month—a big data gap. Mishra hoped a new satellite could give them a clearer picture of coastal health.
They joined forces to create UGA’s Small Satellite Research Laboratory, staffed by about 50 students—ranging from STEM majors to those in business and the arts—and guided by faculty in geography, engineering, math, physics, chemistry and oceanography.
The students first secured NASA funding for a SPOC (SPectral Ocean Color) Satellite. The payload would have optics capable of zooming in on coastal areas and then splitting the light on the water or wetland surface to determine dominant colors. Those colors reveal the chemical and molecular makeup of the ocean and wetland surface and signs of coastal health.
Then they secured funding from the Air Force Research Laboratory’s University Nanosatellite Program, successfully competing against some of the nation’s top established aerospace programs to secure one of two winning spots.
Their second satellite design, MOCI (pronounced mo-chee), or Multi-view Onboard Computational Imager, is designed to take images from low orbit and then compute data before sending it back to ground control. It’s an innovative approach; typically, NASA missions send raw data back to Earth to be processed.
SPOC and MOCI are slated for launch in late 2019 and 2020, respectively. In just three years, UGA’s involvement with space-systems development has transformed from big idea into reality. Even the experts are impressed.
“I’m astonished by how far and how fast they’ve come in a short amount of time,” says Roger Hunter (BS ’78), program manager for NASA’s Kepler Mission, who now manages the Small Spacecraft Technology Program at the Ames Research Center in Silicon Valley.
Adams says the students are inspired by the possibilities this work can support.
“At the end of the day,” he says, “our goal is to support our faculty and create tools that help them do science.”
This story was published originally in the December 2018 issue of Georgia Magazine. The original story is available at https://news.uga.edu/space-dawgs/.
Finding space on the spectrum
By Allyson Mann
Every day we rely on devices—cell phones and computers, for example—that require space on the radio frequency spectrum to carry electrical signals. But the spectrum is running out of frequencies as our dependence on technology increases. Associate professor of engineering Mable Fok, recipient of a National Science Foundation CAREER Award, is using photonics to figure out how to maximize these resources.
What is RF photonics?
RF photonics is the science of using light to process radio frequency signals. Normally when we have a signal, we use a computer or some other electronic component to process it. But it’s getting harder. For example, when we look at movies on our phone, we don’t want any buffering—we want things to be fast. There’s lots of information coming in, and electronics cannot really deal with it. RF photonics is more capable of simultaneously processing a lot of information using light.
How is your work with the glass knifefish connected to your work in photonics?
That particular fish lives in the deep ocean where there’s no light. They can’t see, so they sense their environment by sending out an electric field and receiving it back. Distortion in the electric field tells them if there’s a rock they need to swim around, or if there’s something dangerous they need to avoid, and it also helps them communicate with other fish.
When two of these fish run into each other, what if they’re using a very similar frequency and can’t tell which signal is theirs? They would be confused, which could be dangerous. But when two of these fish emit a similar signal in the same area, the frequency—how fast it’s oscillating—will always repel the other. If the frequencies are too close, they will automatically move away from each other. It’s not really color, but for example, if the first fish uses a red color signal, and the second fish is also using a red color signal, one will move to orange or yellow so they can distinguish each other.
How can photonics help with limitations on the radio frequency spectrum?
Neuroscientists know how the glass knifefish adjusts its frequency to avoid jamming. We want to borrow that neural algorithm and add it to our communication system to allow for more flexibility in how our resources are used. Right now we have the Federal Communications Commission to tell us what frequency to use for your phone, what frequency to use for a GPS system, what frequency to use for a drone, so everything is organized. But we’re not always on the phone, so at certain times those resources are not being used. If we can allocate the resources on demand, based on availability, then more people can use them.
What other uses do you see for light?
Recently we’ve been looking at using fiber optics sensors for soft robotics. Normally our concept of robots is rigid. They don’t really stretch. Over the last couple of years, people have been using soft materials to make robots safer around humans and to perform tasks that traditional rigid robots can’t do. Fiber optics can also be safer in certain environments. If you’re using electronics and hold a wire in a thunderstorm, you probably will get struck. If you’re using an optical fiber, it’s glass, so you won’t get struck. It is immune to electromagnetic interference and any kind of electricity, sparks or lightning. You can use it inside an MRI machine.
Glass fiber is soft, and it’s very flexible, but it cannot be stretched at all so we’ve played around with the architecture. I was inspired by two things—one is the toy my kid was playing with, the Slinky. The other is the DNA structure, which is also spiral. If we put a sensor in a spiral structure, we can easily stretch it, and it’s very reliable.
What would most people be surprised to learn about light?
When I was in grad school, my sister asked me how she should describe my work to her friends. At the time I was measuring things from a laser, and I told her, “I’m doing things that are so small that you can’t really see them. It’s as small as dust.” When I was a postdoc, my adviser pointed out that we don’t work on visible light like red, orange or blue—we work on invisible light. Sometimes we work on pulses so tiny that even the equipment cannot see it. These days I tell my sister that we work on something very small, even invisible, but it has a lot of applications that you’re using every day—for example, the internet. We don’t have fiber optics cable to a house, but the backbone under the ocean uses laser, it’s invisible light. You can’t see it, but you’re using it every day.
Cutting edge water tunnel aids engineering research
The water tunnel will help researchers develop new methods of generating electricity and space and marine vehicles
UGA agrees to establish joint research center with Beijing University of Chemical Technology
The new center will develop new research and enhance existing research
UGA engineering programs earn accreditation
Engineering enrollment has grown from about 400 students in 2012 to more than 2,100 undergraduates and 120 grad students
Engineering students win award in robotics competition
The team taught their robot to harvest ‘apples’
Engineering graduate student receives prestigious research award
Jason Wright will study how geosynthetics perform when incorporated into the design of north Georgia roadways
The art of reduction
Photography by Nancy Evelyn
An ancient Japanese legend says a person who folds 1,000 paper cranes is granted a wish from the gods. For Zion Tse, paper folding—or origami—was how he and his brother entertained themselves growing up in Hong Kong with two working parents.
“Basically, every toy we had was a do-it-yourself out of newspaper. We created paper race cars, paper castles and paper robots,” said Tse, associate professor of engineering. “Because of that, I have been fascinated by the beauty of this Japanese art craft since I was young.”
At Tse’s Medical Robotics Lab, origami provided the inspiration for graduate student Austin Taylor to design a novel cardiac catheter. Working with Tse, Taylor developed a device that’s small enough to fit on the tip of a catheter, but expands once inside the heart to provide physicians with high-quality imaging and therapeutic tools.
Origami techniques have been used in other biomedical applications, but Taylor’s prototype is the first to add imaging capabilities to a catheter. The research was published in the Journal of Medical Devices.
Taylor’s catheter is designed for ablation, a procedure used to treat patients whose hearts may beat too fast, too slow, or erratically. Such arrhythmia prevents the heart from pumping blood effectively and, if untreated, may damage the lungs, brain and other organs. The catheter delivers radiofrequency energy to cauterize the area of heart tissue that’s causing the problem.
His design adds magnetic resonance imaging coils that capture high-resolution scans inside the heart during the procedure. Normally, physicians rely on external MRI coils and use electrical impulses generated by electrode catheters to locate abnormal sites in the heart.
“Placing an imaging coil inside the heart will allow us to locate abnormal tissue more accurately,” said Taylor, who is pursuing a Ph.D. in biomedical engineering. “Combining imaging technology and ablation tools in the same catheter also reduces costs by decreasing the amount of time the MRI machine is used.”

Tse and Taylor’s work is part of a broader engineering trend. Origami techniques have become a popular strategy for reducing the size of objects that need to open up and become bigger. Applications have included heart stents, automobile air bags and satellite solar panels.
The movement began to unfold in the mid-20th century after Japanese origamist Akira Yoshizawa developed universal, picture-based instructions for paper folding that helped bridge the gap between art and science as well as different cultures.
American physicist Robert Lang made further advances, exploring the mathematics underlying the art form and pioneering new design techniques after leaving his original career in 2001 to focus on origami. One project involved a telescope with a lens the length of a football field; Lang calculated how to fold the lens to fit inside a rocket and then reopen in space.
Taylor’s enhanced heart catheter needed similar delivery and functionality, but on a much smaller scale. To create his device, he used an origami shape known as flasher, which is small when folded but expands rapidly. A YouTube instructional video labeled “easiest flasher” involves performing more than 50 folds and pleats. Early versions took Taylor 20 to 30 minutes to create.
“What’s nice about that shape is that when it folds down, the exposed surface area becomes very small compared to when it’s unfolded. We got about a 5-to1 ratio on that size difference for our prototype,” he said. “If we fabricate it at a much smaller level, we can get a 10-1 ratio.”
Taylor, a lifelong athlete whose interest in biomedical engineering was sparked by his own injuries and recoveries, is also finding inspiration in a child’s toy. Using the principles behind Jacob’s Ladder, a toy made of wooden blocks woven together with cloth, he’s creating an endoscopic device that offers increased mobility and control.
Isolating tumor cells
Cells that break away from a cancerous tumor offer a lot of information, potentially leading to new avenues of research, quicker diagnoses and targeted treatments. The challenge is finding these tumor cells and separating them from the billions of other cells circulating in a person’s bloodstream.
UGA researchers collaborating with scientists at several institutions have created a microfluidic device to isolate tumor cells quickly and efficiently. In a paper published in the Royal Society of Chemistry journal Lab on a Chip, the researchers said their technology recovered nearly 93 percent of circulating tumor cells (CTCs) for analysis. Existing, similar devices typically recover only 82 percent.
The new device funnels blood through channels smaller than a human hair. By magnetizing the medium surrounding the cells, the researchers are able to drive cancer cells and blood cells into separate streams based on differences in their size.
“Instead of searching for and enriching the few CTCs that exist in a sample of blood, we’re taking the opposite approach and eliminating everything we are not interested in—the red blood cells, the white blood cells and other components,” said Leidong Mao, senior author and associate professor in the College of Engineering.
Wujun Zhao, a doctoral student in UGA’s department of chemistry, part of the Franklin College of Arts and Sciences, served as lead author.
This brief appeared in the fall 2017 issue of Research Magazine. The original press release is available at http://news.uga.edu/releases/article/new-method-of-isolating-tumor-cells/.
Coatings help medical implants resist infection, clotting
UGA researcher developing coatings that prevent bacterial growth
Helping communities bounce back
By Mike Wooten
Photo: Alan Cressler / U.S.G.S.
As more Americans move to urban areas, forests and agricultural land are often converted to buildings, roads and parking lots. Such development often reduces the amount of rainwater that soaks into the ground and alters the paths it travels to streams and creeks. These changes can leave businesses and homes vulnerable to urban flooding when intense or prolonged rainfall overwhelms the capacity of stormwater systems and floodplains, as it did during Atlanta’s historic 2009 floods.
“Resilience isn’t just resistance to floods or sea level rise,” said Brian Bledsoe, the Georgia Athletic Association Distinguished Professor in Resilient Infrastructure in the College of Engineering.
“A bridge that doesn’t wash away in a flood is resistant, but that’s not all there is to resilience. Resilience is about preparing for and bouncing back from adverse events like floods and hurricanes so that you are less vulnerable and better able to adapt and thrive in the future.”
Urban floodplains, streams, forests and city parks are a few examples of green and blue infrastructure. Gray infrastructure—conventional concrete and piped drainage systems—is designed to quickly move urban stormwater into pipes and downstream. In contrast, green infrastructure like Atlanta’s Historic Fourth Ward Park acts as a sponge or storage area to reduce and treat stormwater at its source while providing recreational, aesthetic, health and other benefits.
“By working at the intersection of designed natural systems and the built environment, we can make our infrastructure more resilient while generating numerous environmental, social and economic benefits,” said Bledsoe.
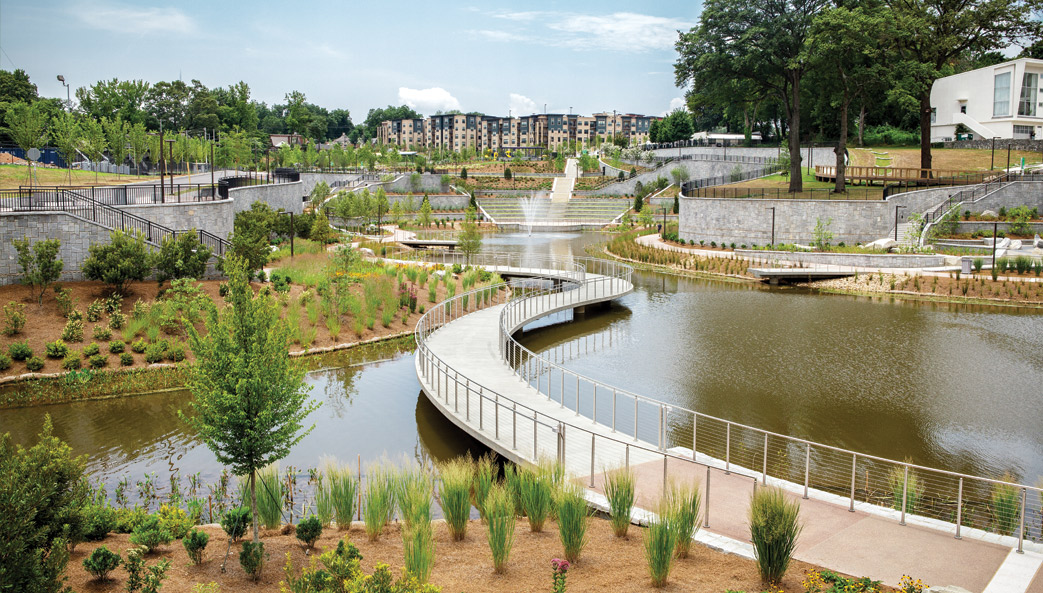
Infrastructure that works with nature
To help communities adapt their infrastructure in a time of rapid environmental and social change, Bledsoe is leading a new cross-disciplinary research initiative at UGA. The Institute for Resilient Infrastructure Systems (IRIS) will explore ways to integrate green and blue infrastructure with traditional gray infrastructure systems. In addition to the College of Engineering, IRIS includes nearly 30 faculty members from eight other academic units across the UGA campus.
“IRIS is unique in that it unites engineering with ecology, environmental design and planning, atmospheric science, law and policy, public health, economics and other disciplines to effectively integrate green and gray infrastructure solutions for resilience to weather and climate-related extremes,” said Bledsoe.
In addition to working with cities on problems related to their aging infrastructure, IRIS will look to help communities along the coast as they grapple with sea level rise, and rural areas as they face water scarcity and other challenges.
“The challenges facing the nation’s infrastructure go well beyond potholes, broken pipes and traffic jams,” said Bledsoe. “We need to make significant investments in infrastructure, but we need to integrate the gray with the green and blue in a way that enhances our well-being and builds healthier communities.”
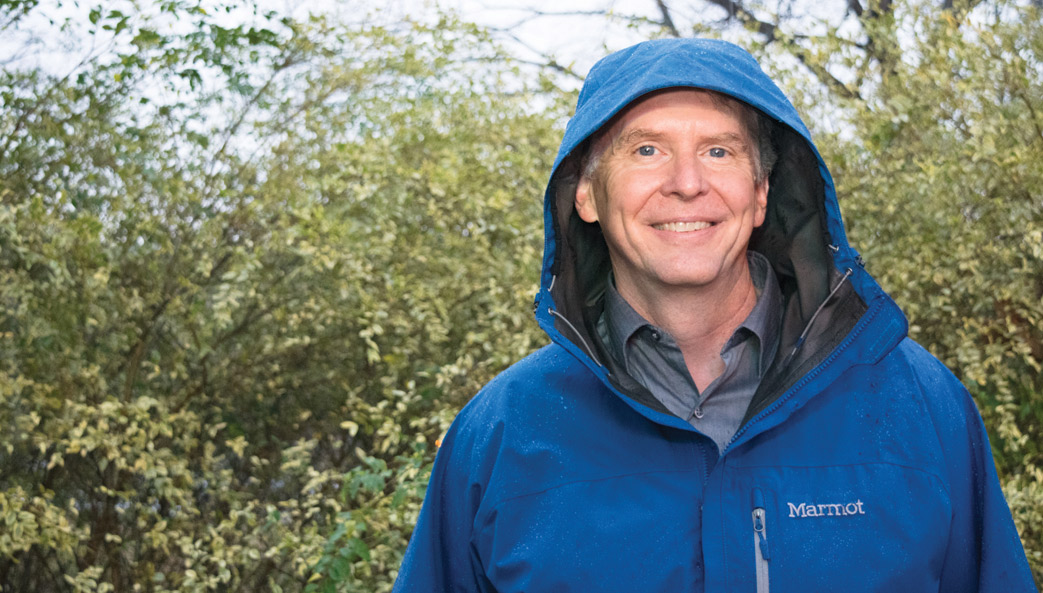
Researcher explores how light can solve wireless space shortage
Signals from wireless devices are crowding an already limited number of available radio frequencies.
Transformers: Robots and drones accelerate plant genetic research
High-tech tools commonly found in factories and on the battlefield may soon play a major role in helping feed the world’s rapidly growing population.
UGA researchers are developing a robotic system of all-terrain rovers and unmanned aerial vehicles that provide quick and accurate phenotyping—gathering and analyzing data on the physical characteristics of crops, including their growth patterns, stress tolerance and general health. Such information is vital for scientists working to radically increase agricultural productivity in a time of rapid population growth.
“By the middle of this century scientists estimate the world’s population will reach 9.1 billion people, which is a 30 percent increase in a little more than 30 years,” said Changying “Charlie” Li, professor of engineering. “This increase in population will demand that we nearly double our current food production. That’s a tall order, but one solution is to use genomic tools to develop high-quality, high-yield, adaptable plants.”
The current process for gathering data on plant characteristics is expensive and painstakingly slow, as researchers must manually record their observations one plant at a time. But the team of robots developed by Li and his collaborators will one day allow researchers to compile data on entire fields of crops throughout the growing season.
The project addresses a major bottleneck that’s holding up plant genetics research, said Andrew Paterson, Regents’ Professor and leader in mapping and sequencing flowering-plant genomes.
“The robots offer us not only the means to more efficiently do what we already do, but also the means to gain information that is presently beyond our reach,” he said. “For example, by measuring plant height at weekly intervals instead of just once at the end of the season, we can learn about how different genotypes respond to specific environmental parameters, such as rainfall.”
In addition to multispectral, hyperspectral and thermal cameras, the robots will be outfitted with Light Detection and Ranging, or LiDAR, a remote sensing method that uses light in the form of a pulsed laser to measure distances. The technology will allow the researchers to create precise three-dimensional images of plants.
During preliminary testing last year, the team collected an estimated 20 terabytes of data over the six-month growing season. Li said the team will collect 30 times that amount when the robots are fully deployed.
To analyze these massive data sets, the researchers are developing an artificial intelligence algorithm similar to the facial recognition program Facebook uses to facilitate the identification and “tagging” of people in photographs.
“Our algorithm will be able to scan an aerial photo of a large field and automatically identify the location and number of flowers on each plant,” Li said.
With fleets of autonomous vehicles rumbling through rows of crops and hovering overhead, algorithms also will play a key role in making sure the robots and drones perform their assigned tasks while staying out of each other’s way. Javad Mohammadpour Velni, assistant professor of engineering, is developing a suite of analytical tools that will allow the ground and aerial vehicles to operate independently but collaboratively to efficiently cover fields and collect data.
The researchers believe their work will provide a platform for plant geneticists to gather large amounts of data and empower advances in crops to sustain the planet’s population.
“Historically, genetics has been credited for about half of the yield gains that permitted small numbers of farmers and producers to sustain large human populations, for example during the Green Revolution,” Paterson said. “It’s realistic to envision that genetics will need to account for about half of the doubling of agricultural output that’s needed by 2050. This will require roughly doubling historical rates of progress in crop improvement, and more detailed and efficient phenotyping will be essential.”
This story appeared in the fall 2017 issue of Research Magazine. The original press release is available at http://news.uga.edu/releases/article/robots-and-drones-improve-crop-yield/
Tightrope robots
A robot invented by researchers in UGA’s College of Engineering could change the way power lines are inspected—providing a safer and more cost-effective alternative.
Tightrope robots
A robot invented by researchers in UGA’s College of Engineering could change the way power lines are inspected—providing a safer and more cost-effective alternative.
UGA researchers unfold advances in cardiac catheters
A graduate student in the University of Georgia College of Engineering is turning to the ancient Japanese art of origami for inspiration as he designs a novel cardiac catheter.
Inside information
May 10, 2016
Researchers at the UGA College of Engineering are working to bring greater accuracy to the navigation systems used to guide unmanned aircraft known as drones.
The team, which is led by assistant professor Zion Tse and includes postdoctoral research fellow Stan Gregory and graduate research assistant Kevin Wu, has designed and tested a prototype that employs ultrasound technology to help drone operators map the interior of large structures and guide their craft to specific locations within it.
Conventional “GPS technology isn’t a good option for what we’re trying to accomplish because it’s not very reliable indoors,” Wu said. “The margin of error can be up to 5 meters, which is fine if you’re driving your car and dealing with a scale of miles.” But when drones are inside a building, he said, “they need a much higher degree of precision.”
In much the same way a bat navigates in dark spaces, the system’s onboard ultrasound sensors relay information on the drone’s location to a computer, which plots the area and tracks the device’s position for the benefit of the operator.
The team’s work is supported by the Southern Company, one of the nation’s largest energy firms, which plans to use unmanned aircraft to enhance safety for crews in the field and improve reliability for customers. Thus, according to Gregory, he and his colleagues will test their guidance and mapping system for the first time this spring at Southern Power’s Plant Franklin on the Alabama-Georgia border in Smiths, Alabama.
Southern Power is a subsidiary of the Southern Company, and Plant Franklin is designed to capture waste heat from its primary combustion turbines and use the heat to increase the plant’s efficiency. This process happens in an area known as a heat recovery steam generator. Plant Franklin’s three heat recovery steam generator units are 20 feet long, 20 feet wide and reach 70 feet high. The inside of each unit is covered with large, specially treated steel plates.
During traditional maintenance-related plant outages, when the heat recovery steam generator units are inspected, workers construct temporary scaffolding so that inspectors may climb along the inside walls to look for potential problem areas.
The team’s system is designed to streamline this process. “The inspection drone will reduce cost and plant downtime by allowing a single inspector to assess the recovery system without the need for scaffolding,” Tse said.
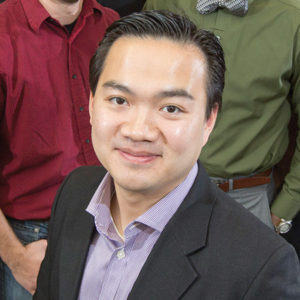
Zion Tse is an assistant professor in UGA’s College of Engineering.
UGA team selected by NASA, Air Force to build and launch two cube satellites
The team will receive $200,000 in funding to prepare for a launch 18 months from the project start date this month.
Robot developed by UGA engineers offers safer, more efficient way to inspect power lines
A robot could change the way power lines are inspected—providing a safer and more cost-effective alternative.